PROJECTS
Thinking across Scales
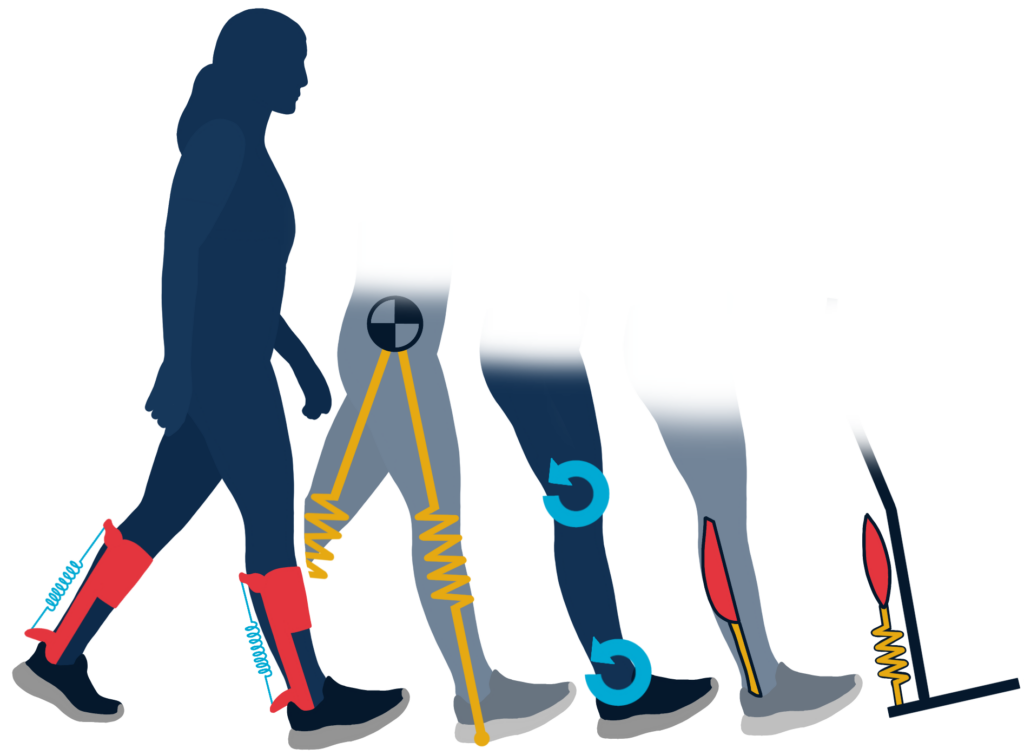
Figure by Jenny Leestma
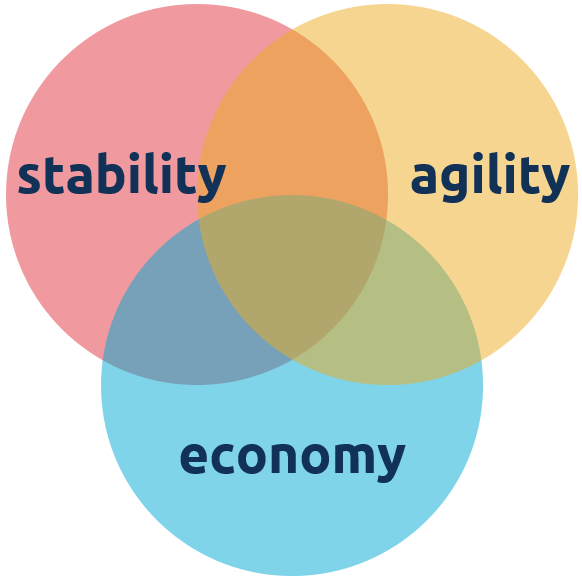
Active Projects
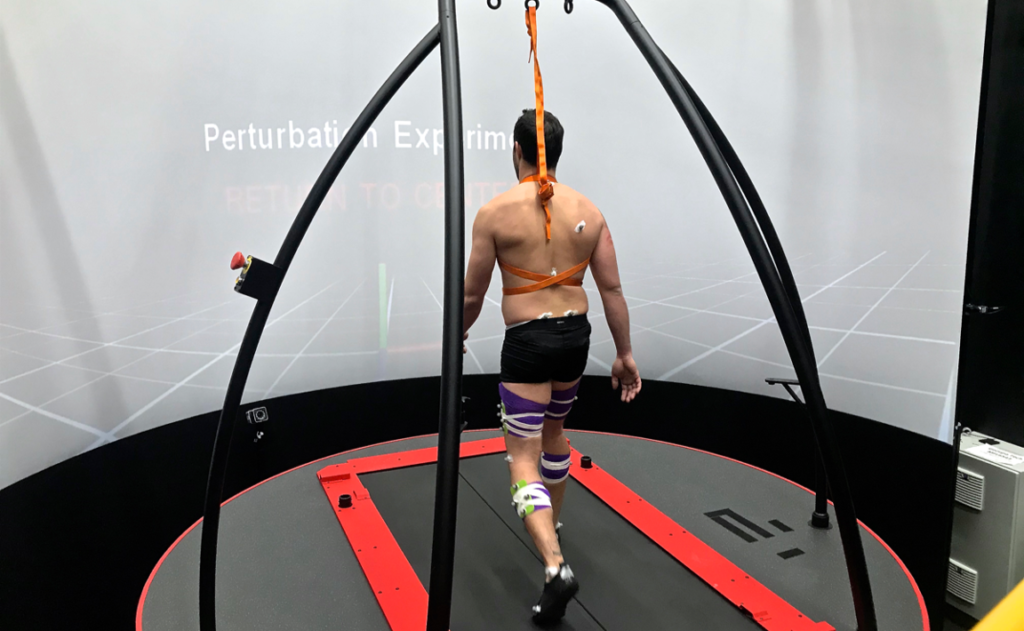
Balance augmentation
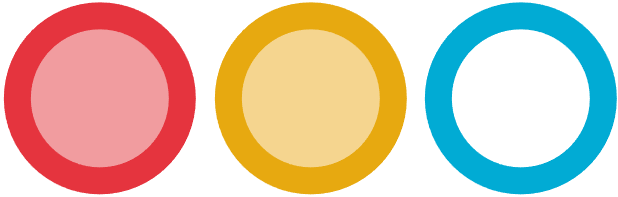
To build wearable devices that accommodate users throughout non-uniform terrain in the world outside of the lab, we must first understand the biomechanics of non-steady state locomotion. Using our lab’s CAREN system, we are able to rapidly move the locomotion platform in 6 degrees-of-freedom, allowing for the application of sudden perturbations or continuous unstable environments. We are investigating biomechanical and neural control responses during unstable tasks through the collection of motion capture, ground reaction forces, and electromyography (EMG) data. The results from these experiments will be used to inform the design and control methods for a wearable hip exoskeleton that augments human stability during challenging locomotion tasks.
Lab Members: Sofia Arvelo Rojas, Theresa Hardin, Dr. Kristen Jakubowski
Collaborators: Dr. Aaron Young, Dr. Lena Ting, Dr. Jennifer Leestma
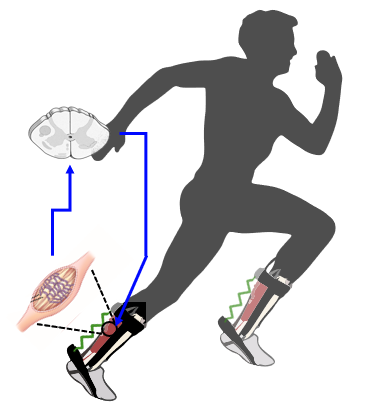
Exoskeleton effects on sensorimotor feedback and control
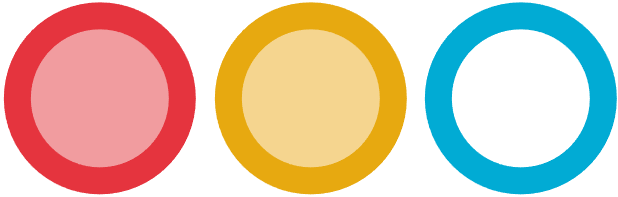
Exoskeletons act mechanically in parallel to a joint and have been shown to affect muscle dynamics and kinematics in significant ways, in turn affecting overall muscle stiffness and activation and the proprioceptive organs within. The feedback we gain from these proprioceptive organs contributes to overall movement control and stability. Although significant advancement in wearable technologies for assisting locomotion and augmenting balance, little is known about this interaction between these exoskeletons and our internal sensorimotor control system. We aim to rigorously identify the effects of these assistive exoskeletons on the sensory feedback humans experience and their impact on sensorimotor control on different timescales. We look at both short- term responsiveness and long-term accuracy during agile, volitional movements and perturbed, stabilizing movements. With this work, we extend unsteady, muscle-tendon benchtop experiments in animals to humans in “real-world.”
Lab Members: Amro Alshareef, Dr. Hansol Ryu
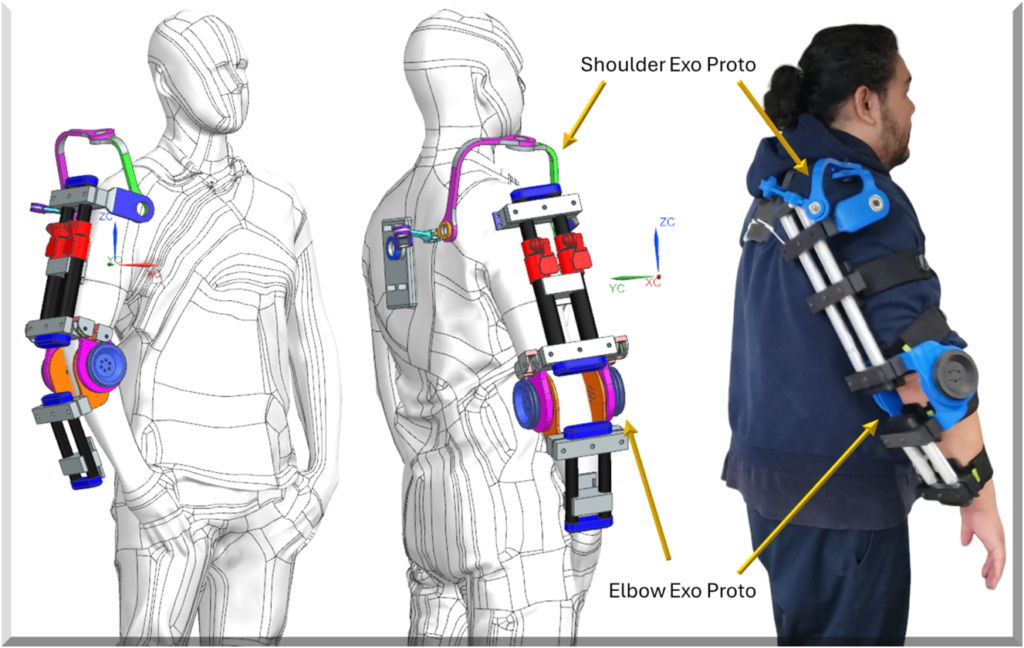
Task Agnostic System Architecture Development of an Upper Body Exoskeleton for Occupational Tasks
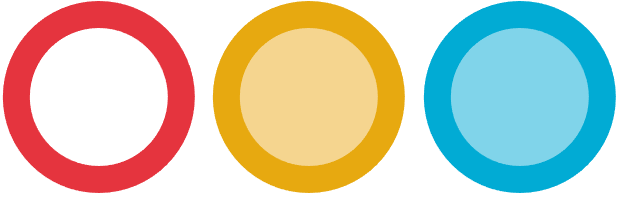
The upper body team’s focus is to develop upper body wearable devices that implements smart controllers based on biofeedback markers. As such, our team is heavily involved in the following: (1) human subject collection and analysis of occupational and/or activities of daily living (ADL) task biomechanical data for upper body motions, (2) Designing hardware for multi-degree of freedom (DOF) upper limb exoskeletons and prothesis, (3) Implementation of intelligent control architectures using machine learning that utilize the data from (1) into (2).
Lab Members: Carlo Canezo
Collaborators: Dr. Divya Srinivasan, Dr. Robert Griffin, Dr. Gwen Bryan, Jared Li
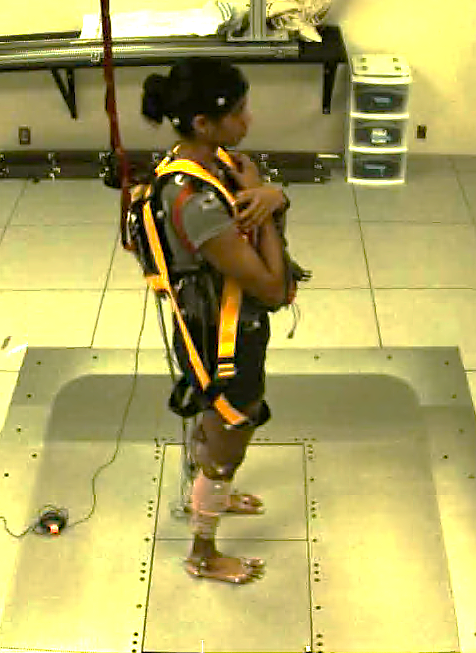
Walking and standing balance in pregnancy
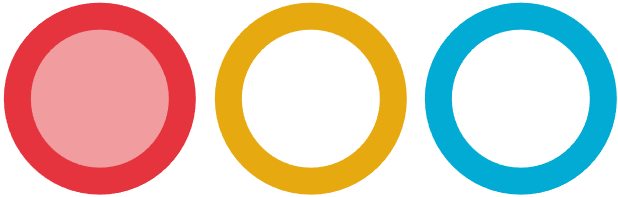
Falls in pregnancy are common and dangerous, but their causes are largely unknown. Potential factors such as tissue remodeling, maladaptation to added mass, and increased fear of falling, are difficult to separate. We are isolating the variable of added mass by asking non-pregnant participants to wear weighted backpacks in different orientations on their torso. We can then assess changes in balance ability with added mass during standing (on the Neuromechanics Lab’s standing balance platform) and walking (on the PoWeR Lab’s CAREN system). Next, we will collect the same data in pregnant participants to compare with our weighted controls.
Lab Members: Autumn Routt, Dr. Kristen Jakubowski
Collaborators: Dr. Lena Ting
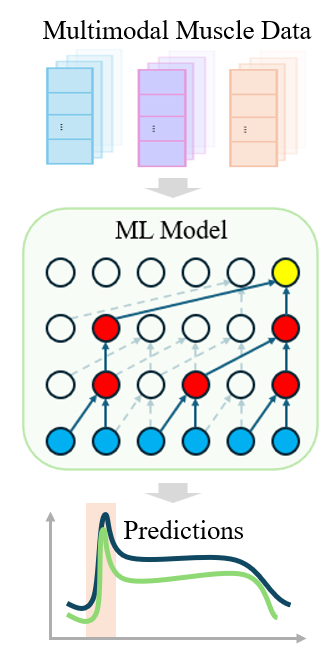
Data-driven muscle modeling in perturbed conditions
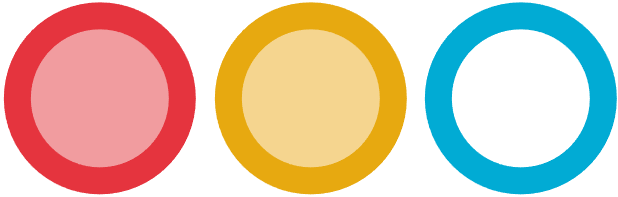
Muscles are critical components for animal locomotion. Muscle modeling helps us understand muscular mechanisms and provides inspiration for wearable robotics. However, current muscle models struggle to predict muscle responses under perturbed and dynamic conditions, which limits the application of wearable robotics in real-world scenarios outside the laboratory. Using our lab’s biorobotic interface and dynamic dynamometer, we can emulate perturbed and dynamic movements to study muscle responses in both animals and humans while recording physiological data through various methods including sonomicrometry, ultrasound imaging, and EMG. These multimodal datasets are then used to train and develop machine learning-based muscle models. The results from this project will advance the development of more naturalistic wearable robotic systems.
Lab Members: Shu Gong, Amro Alshareef, Dr. Hansol Ryu
Collaborators: Dr. Tim Cope, Dr. Lena Ting, Dr. Laksh Punith
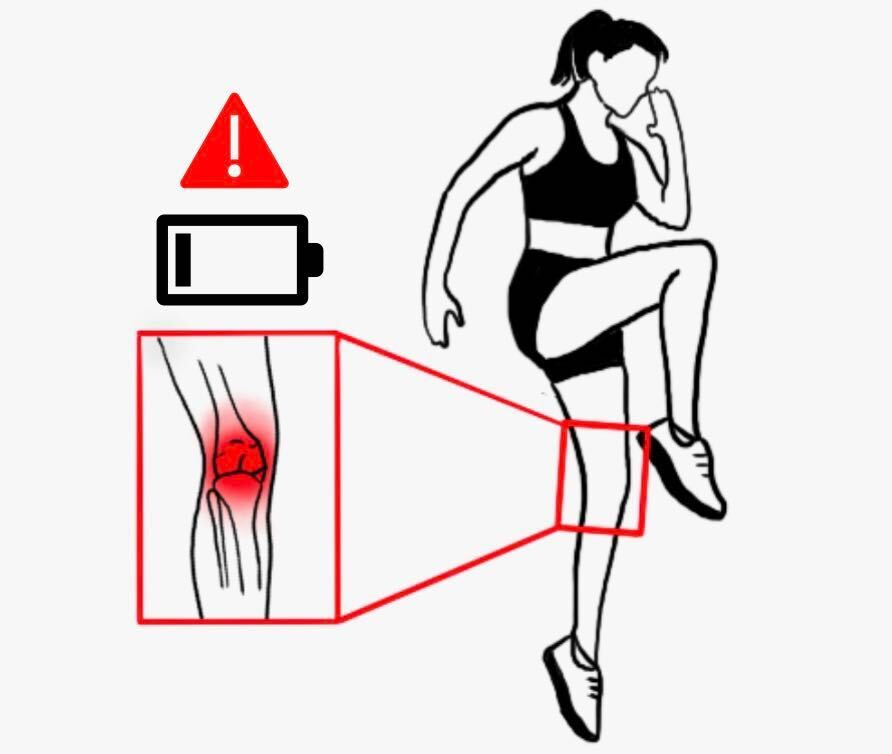
Impacts of Neuromuscular Fatigue on Motor Coordination
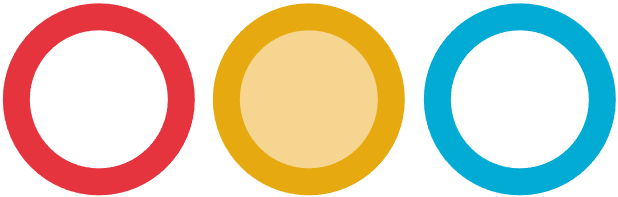
Neuromuscular fatigue is a highly task-dependent condition that is known to have significant influences on neuromuscular and biomechanical properties in humans. Despite these potential outcomes, task performance can be maintained for long periods by leveraging motor redundancies to compensate for the aforementioned fatigue-induced changes. The central aim of this research is to characterize the role of neuromuscular fatigue in informing strategies of motor coordination of the lower limb.
Lab Members: Melody Modarressi
Collaborators: Dr. Young-Hui Chang
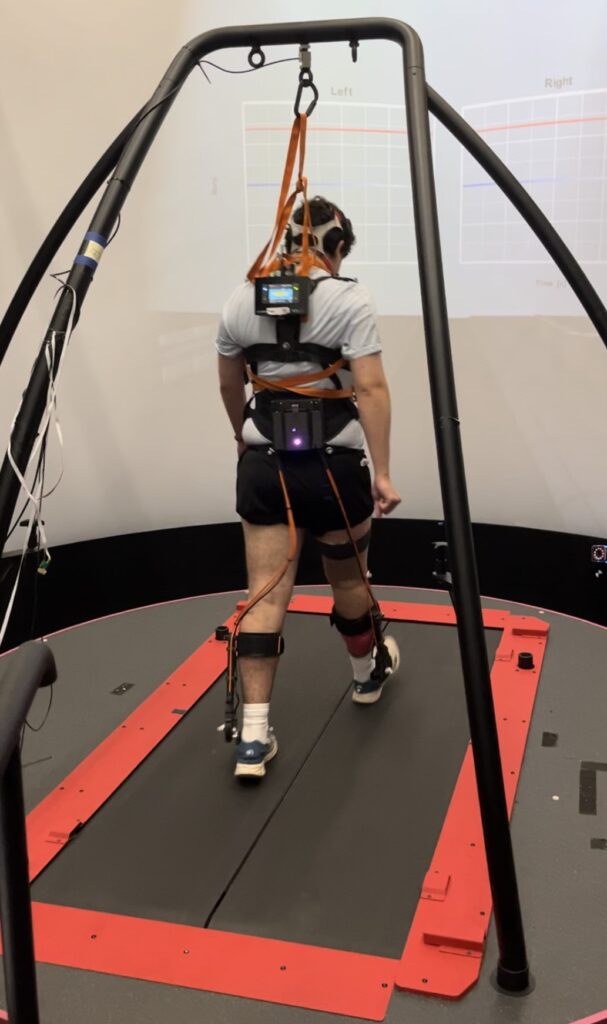
ExerExo: Exploring the effects of assistive and resistive ankle exoskeleton torque on walking mechanics and energetics
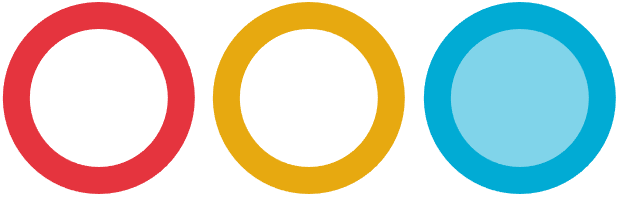
This project looks to explore the efficacy of ankle exoskeletons as wearable exercise training devices by examining the mechanics and energetics of walking. The exoskeleton will be used to provide walking assistance and resistance at various walking speeds in the form of positive or negative plantar flexion (push-off) torque. Walking with exoskeleton resistance simulates increased exercise volume and effort, while assistance reduces exercise volume and effort. The project will look to determine changes in biological ankle torque, kinetics/kinematics, muscle activation, metabolic activity, and heart rate across simulated levels of walking exercise.
Lab Members: Arshad Mandani
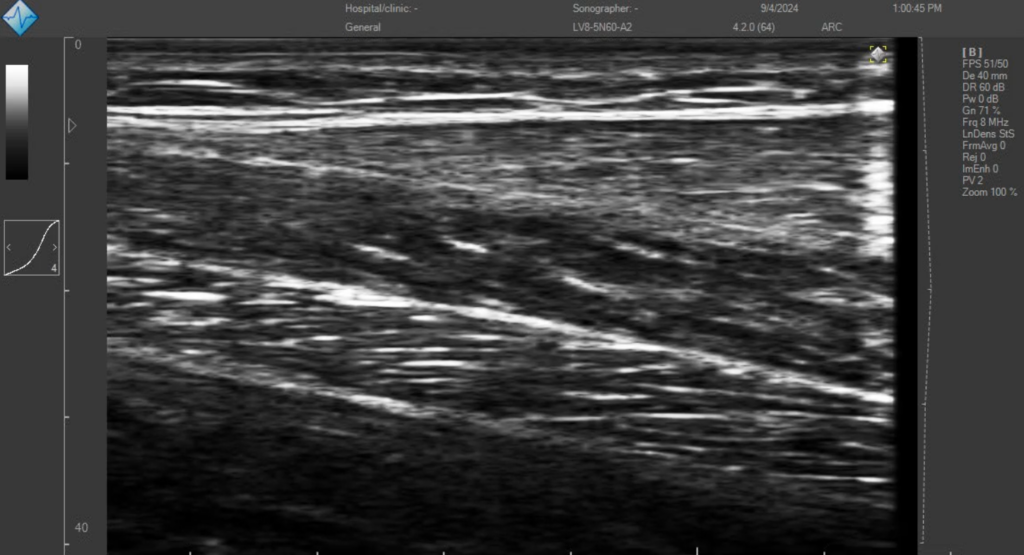
Acute Stretching Effects on Muscle-Tendon Mechanics
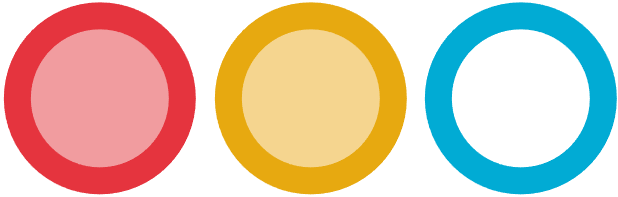
In this project we aim to understand how stretching affects the viscoelastic properties of muscles and tendons. Previous work has shown that muscles and tendons will change stiffness when stretched, but there is a gap in the literature regarding the exact mechanism of stretching. This project aims to use ultrasonography to observe muscles and tendons in real time to obtain a better understanding of the stretching mechanism. The viscoelastic properties of the muscle and tendon are highly influential on locomotion strategies, particularly during motions that challenge stability and agility. Interventions like stretching may change these properties, altering an animal’s ability to navigate locomotor challenges. The results of this study will inform musculoskeletal models that capture the dynamic nature of muscle and tendon properties.
Lab Members: Skylar Taylor
Collaborators: Dr. Young-Hui Chang
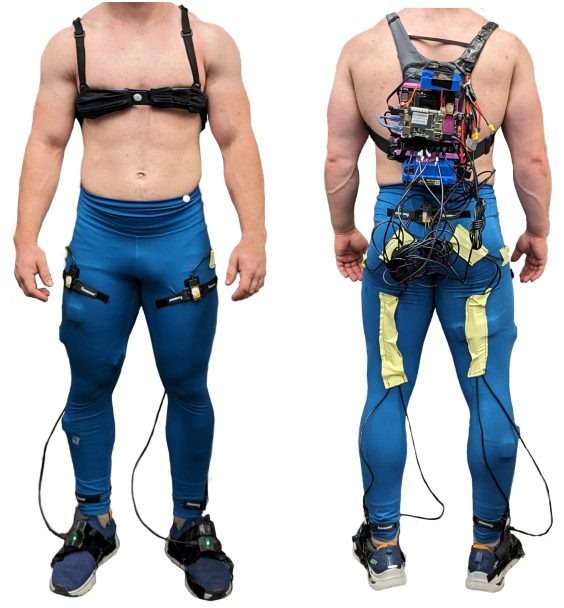
Personalized Exoskeleton Assistance for Enhancing Post-Stroke Mobility and Community Ambulation
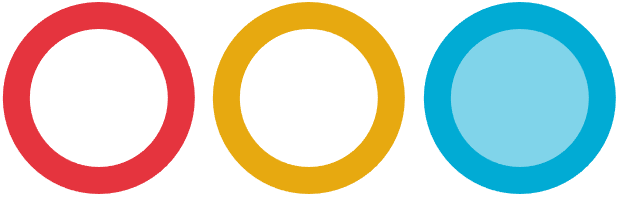
The study focuses on personalized exoskeleton systems targeting ankle and hip joints to optimize gait mechanics and improve walking economy post-stroke. The research aims to compare the effectiveness of hip vs ankle exoskeletons with AI-based controllers and evaluate these technologies in real-world environments to ensure clinical relevance and user adoption. The ultimate goal is to create adaptive, user-friendly exoskeletons that support long-term community ambulation for stroke survivors.
Lab Members: Dr. Daniel Rodriguez-Jorge
Collaborators: Hangyeol Song, Yash Mhaskar, Dongho Park, Dr. Aaron Young
Past Projects
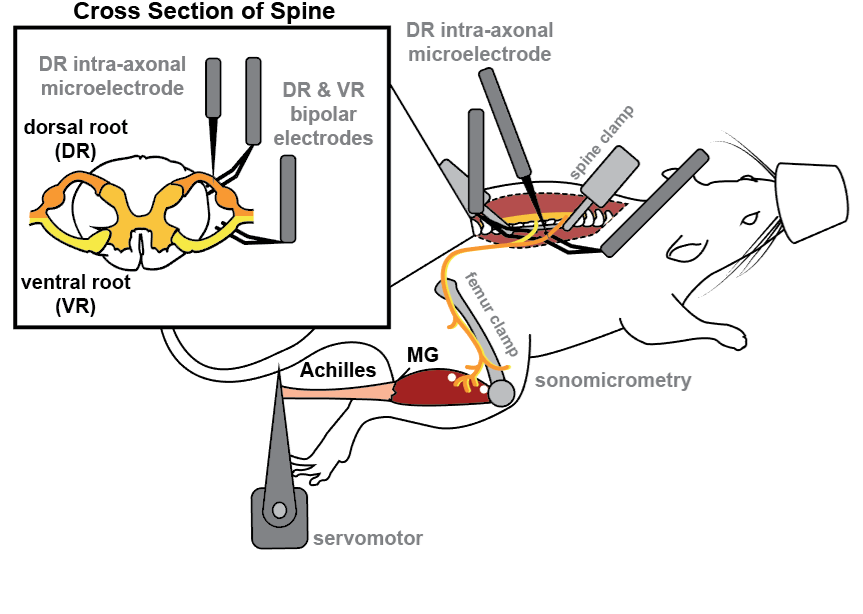
Virtual mechanical reality for muscles
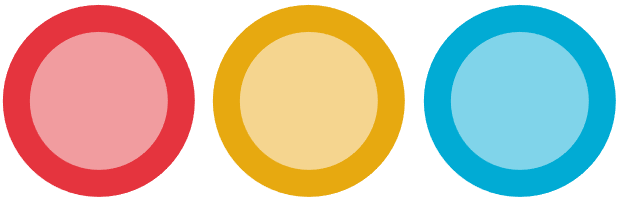
Animals generate movement using the interaction of their musculoskeletal and nervous systems with the physics of their environment. Traditionally, these interactions have been studied by measuring muscle states in freely moving animals or in mathematical models. While animal experiments generate data in the context of interactions with real world physics, it is very difficult to experimentally control their neural stimulation and body properties to test hypotheses. Mathematical models on the other hand lend them selves to complete control, however are currently lacking in their ability to mimic muscle function. Thus our lab combined the best of both worlds and developed a novel ‘virtual mechanical reality’ system for muscles that allows experimenters to connect real muscle-tendon units to a motor that can be programmed to emulate a physical body and environment of choice. We’re using this technology to study how animals navigate uneven terrain, how changes in morphology affect proprioception, and how wearable robots augment the function of muscles during movement.
Lab Members: Dr. Laksh Punith, Amro Alshareef
Collaborators: Dr. Emily Abbott, Dr. Tim Cope, Dr. Lena Ting
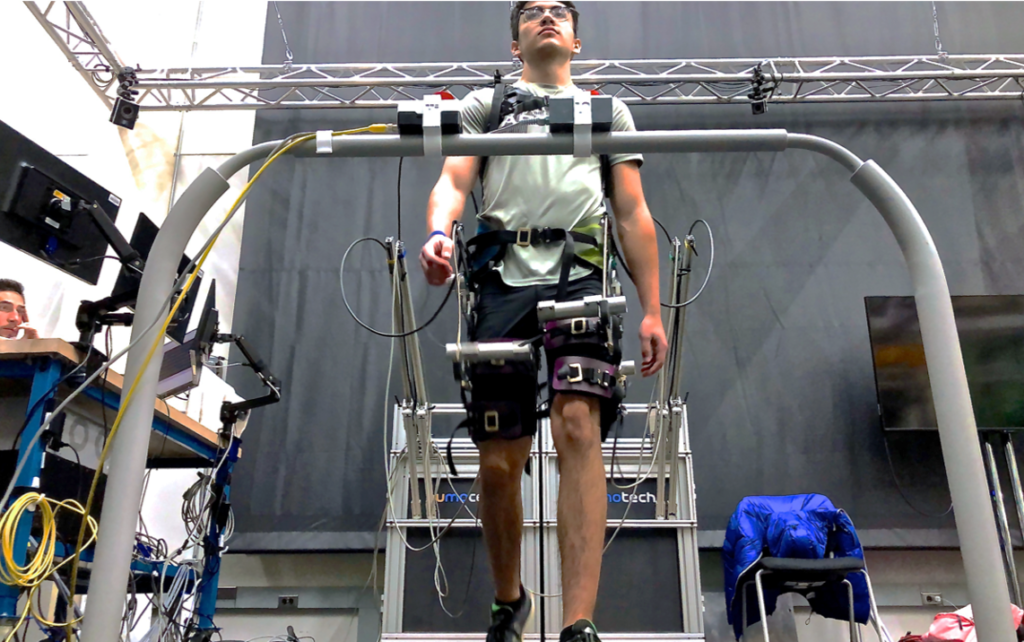
Online optimization of exoskeleton assistance for real-world mobility
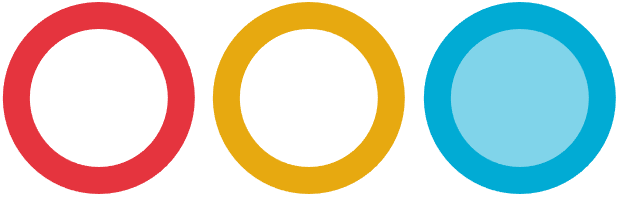
We must tune torque assistance to each user and goal to effectively enhance and understand how wearable robotics affect mobility. Currently, we are applying human-in-the-loop (online) optimization across walking performance outcomes in two ways. First, we’d like to understand if in-lab performance outcomes translate to out-of-the-lab overground walking. Second, we studying why people change their gait with age and when walking with assistance.
Lab Members: Ben Shafer
Collaborators: Dr. Aaron Young
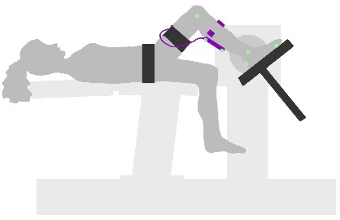
Long-term impact of exoskeletons
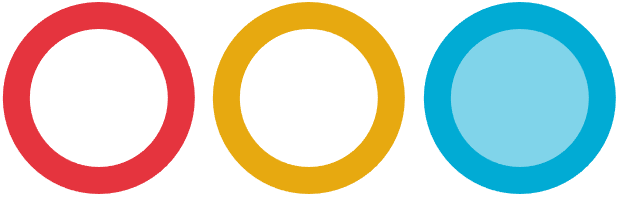
The objective of this study is to investigate musculoskeletal and neuro-mechanical adaptations to frequent wearable device use. Probing impacts of both unloading and behavioral changes can give insight to ‘real-world’ adaption and feedback systems. To study structural and functional adaptations, our lab uses musculoskeletal modeling and human experiments incorporating indirect calorimetry, electromyography, ultrasonography, and 3D motion capture. Understanding effects of long-term exoskeletons use can inform user settings as well as device design and control.
Lab Members: Jordyn Schroeder, Dr. Owen Beck
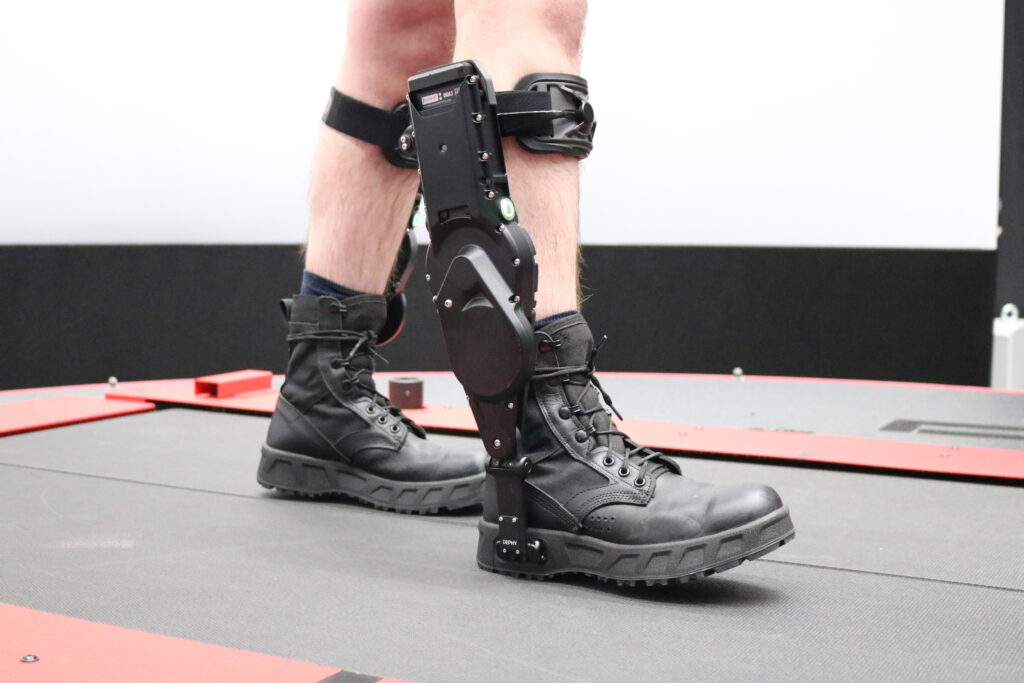
Steering MTU dynamics in aging using exoskeletons
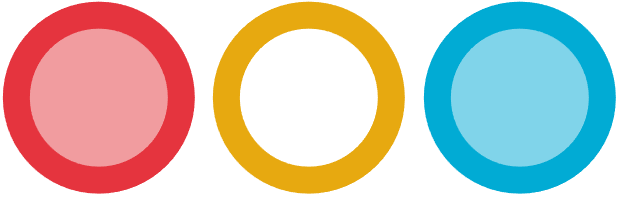
In order to prescribe exoskeletons we must first find a measurement that scales with exoskeleton assistance. Currently, we study older adults to look at changes in muscle force-length curves and tendon morphology to determine if physiology influences their metabolic response to ankle exoskeletons. This also allows us to test if exoskeleton assistance optimized for young adults work for older adults. We hope this work will lead to exoskeletons to keep older adults independent that can be prescribed based on their muscle or tendon properties.
Lab Members: Lindsey Trejo, Amro Alshareef
Collaborators: Dr. Jason Franz
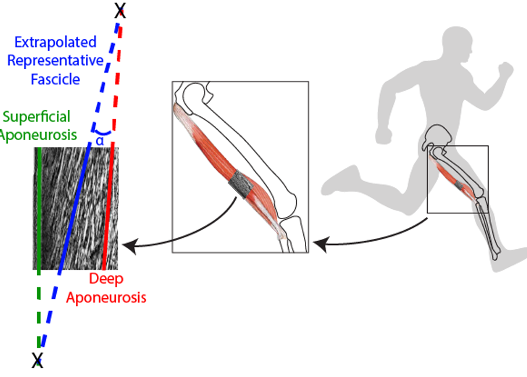
Hip muscle dynamics during locomotion
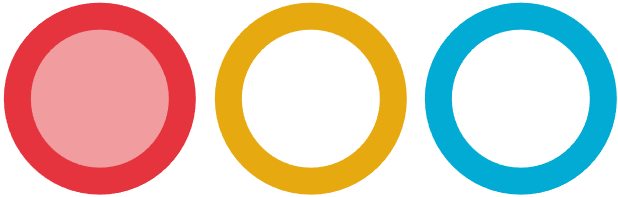
The objective of this project is to directly measure rectus and biceps femoris muscle dynamics during a variety of tasks, like walking, running, and slips. The hip joint is critical for stabilizing walking through proper foot placement and is considered the “motor” of the legs. Thus, understanding how hip muscles produce and transfer energy across the limb can provide a roadmap for the design of assistive devices that work in concert with these major muscle groups to make walking more stable and less metabolically costly. To measure muscle dynamics our lab uses B-mode ultrasound in addition to the biomechanical toolkit of electromyography, motion capture, and musculoskeletal simulations.
Lab Members: Dr. Pawel Golyski
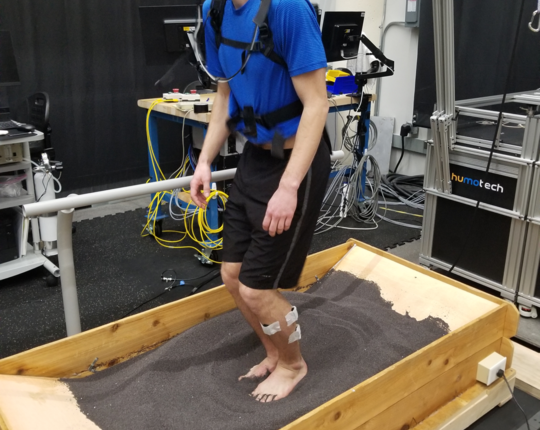
Locomotion in deformable and dissipative terrain
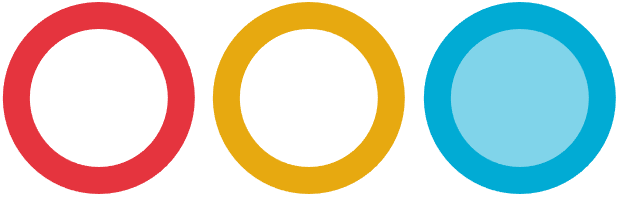
The field of wearable robotics is advancing quickly, however, most devices still struggle in environments with complex terrain such as sand, snow, mud or dirt, which are challenging due to the non-linear physics of interaction between the human-machine system and the environment. We are working to evaluate biomechanics during movement on such terrains and engineer a wearable device that uses a bio-inspired approach to characterize and augment human locomotion over complex media. The insights gained through this project will allow us to create more robust devices and more optimal control schemes that are better suited to “real-world” use of wearable robotics.
Lab Members: Jonathan Gosyne
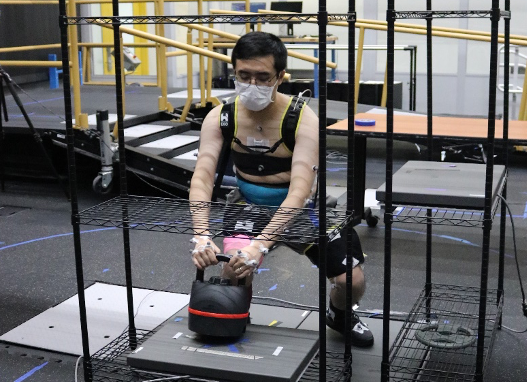
Biomechanics and augmentation of manual labor tasks
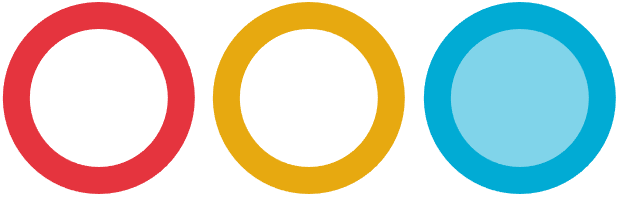
Many workers in hazardous environments and other worksites must regularly perform physically exhausting tasks while wearing heavy protective gear, overexertion from which can sometimes result in debilitating physical injuries. To help increase worker performance and reduce strain on potentially injury-prone joints, we are investigating how to leverage ankle, knee, and lower back exoskeletons to provide targeted assistance during a variety of walking and lifting tasks. The results of this work will help us to determine which exoskeletons are most useful for these kinds of tasks, and will further inform our development of more intuitive and effective exoskeleton control strategies down the road.
Lab Members: Felicia Davenport, Jennifer Leestma
Collaborators: Dr. Aaron Young, Dr. Anirban Mazumdar, Josh Fernandez, Dr. Krishan Bhakta, Raymond Kim, Christoph Nuesslein, Ryan Casey, IHMC Robotics, Sandia National Labs
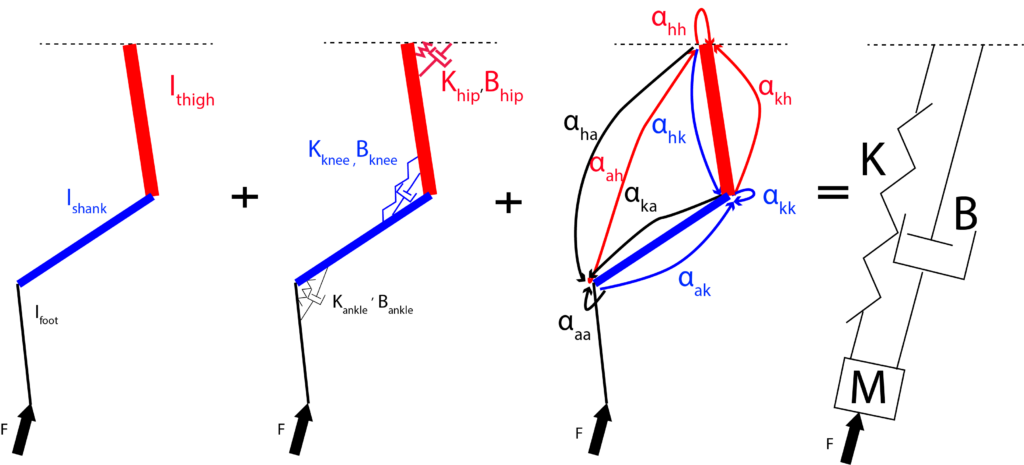
Limb impedance and inter-joint coordination
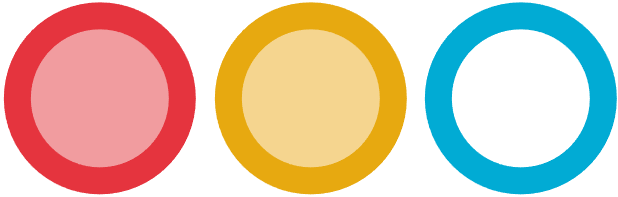
Neural feedback pathways from Golgi tendon organs, which measure active contractile force, are widely distributed in the limb. The firing of muscle spindles is nonlinearly related to length and velocity, and some spindle pathways are inter-joint and asymmetric. Proportional coordination, or equal joint angle excursions, between the knee and ankle has been observed in cats in many contexts despite differences in impedance between the joints. We’re investigating how inter-joint reflex feedback modulates limb impedance and inter-joint coordination across tasks and conditions. Understanding the role of reflex feedback in regulating limb mechanics will inform therapeutic and rehabilitation techniques for stroke and SCI patients.
Lab Members: Thendral Govindaraj
Collaborators: Dr. Richard Nichols